Arterial Compression Technology for a Watch-type Blood Pressure Monitor
- Blood pressure monitor
- Arterial compression technology
- Cuff
- Oscillometric methods
- Pulse sensing
OMRON HEALTHCARE Co., Ltd., is working on the development of a watch-type wearable blood pressure monitor that makes it possible to measure blood pressure anytime, anywhere. The increase in measurement opportunities from the realization of a wearable blood pressure monitor can expect contributions to further hypertensive pressure medical treatment because it makes it possible to continuously catch one-day blood pressure fluctuations. In order to be able to wear it at all times, it is necessary to make the cuff (bladder) width half of the conventional model. When the cuff width is reduced, there is a problem that blood pressure measurement accuracy deteriorates because the higher internal pressure of the cuff must occlude an artery, and the correct pulse waveform cannot be detected. Conventionally, only one cuff carries out the gradual pressurization of an artery in the wrist and detection of the cuff internal pressure, including the minute pressure vibrations that occur from the pulsation of blood pressure. The new cuff structure carries out pressurization of the wrist and detection of the real pressure being applied to the pressed wrist by another cuff. It enables a cuff width of 25 mm and a width less than half of the conventional 52 mm. The company acquired medical equipment certification for the first time in the world and let the development of the wearable blood pressure monitor that guaranteed measurement accuracy succeed.
1. Introduction
1.1縲Background to the development
Modern clinicians recognize the significance of home blood pressure monitoring in the treatment of hypertension and the management of blood pressure. Home blood pressure measurements have become a wide-spread practice, owing to, among other factors, the development of oscillometric blood pressure measuring methods requiring no special knowledge or techniques and the establishment of hypertension diagnosis criteria based on the findings from clinical studies supporting the clinical value of home blood pressure measurements, such as the Ohasama Study and the accumulated results of epidemiological studies 1)–3).
Meanwhile, there are many known cases where sufficient antihypertensive treatment was not provided even after home-use blood pressure monitors became widely available for hypertension diagnosis and treatment4) . One of the possible causes is that several blood pressure measurements taken at home in, for example, the morning and evening or those taken during a consultation at a hospital may not necessarily be sufficient to detect the higher portion of the blood pressure of a person that may fluctuate widely during the 24 hours of the day5) .
Some devices, such as 24-hour ambulatory blood pressure monitoring (ABPM) devices, can be worn all day long to measure blood pressure at 30-minute to 2-hour intervals. Such a blood pressure monitor, however, consists of a cuff wearable around the upper arm and a main unit wearable around the waist and connected via a rubber tube to the cuff, which considerably limits the free mobility of the user. It is difficult to spend every day wearing such a device all day long.
Therefore, OMRON HEALTHCARE Co., Ltd., developed a wearable blood pressure monitor that can be worn all the time without much of a burden on the user to take blood pressure measurements any time as part of continued efforts to contribute further to hypertension diagnosis and treatment.
1.2縲Selection of the blood pressure measuring method
Some recent models of smartwatches and other wearable devices come with a cuff-less blood pressure meter. We, however, decided to develop the blood pressure monitor to be described below, considering it highly meaningful at present to make an existing cuff-based blood pressure monitor wearable because it is recognized as effective in the diagnosis and treatment of hypertension and approved as a medical device.
The cuff-less blood pressure measuring method converts the amount of change in a blood pressure change-related feature amount, such as arterial blood flow rate or pulsation size, into the amount of blood pressure change to obtain blood pressure estimates. This method, however, requires frequent measurements of the reference blood pressure by another blood pressure monitor to ensure measurement accuracy. The reason is that the blood pressure change-related feature amount is affected by the change in hardness or elasticity of the arteries and because this change in the arteries is controlled by the autonomic nervous system and occurs all the time due to various factors in daily life, such as emotions, exercise, meals, sleep, and temperature.
Even after this challenge to the operating principle is overcome, another challenge awaits the cuff-less blood pressure monitor: the device must win recognition among the medical community and those needing blood pressure measurement as comparable to or more clinically useful than conventional blood pressure gauges through demonstrative use in the diagnosis and treatment of hypertension.
After the launch of its first home-use blood pressure monitor in 1978, OMRON Corporation needed 37 years to have the Japanese Society of Hypertension admit in its Guidelines for the Management of Hypertension 20146) that a diagnosis based on home blood pressure data shall prevail when it conflicts with another diagnosis based on clinic blood pressure data. It is expected to take much less time for clinical evidence building and recognition of an innovative wearable blood pressure monitor that will come into being in the future to outperform and take the place of cuff-based monitors. Still, it is unlikely for that time to come within several years. Hence, we consider that development based on the currently accepted measuring method is the quickest way to contribute to the diagnosis and treatment of hypertension.
Nevertheless, the development of a much less restrictive device able to take accurate blood pressure measurements any time will make blood pressure management accurate and easy and contribute directly to solving the social challenge of preventing hypertension-induced cerebral and cardiovascular disease events. We still have a need to continue efforts to develop innovative blood pressure measuring methods and devices.
1.3縲Target cuff width and technical approach
For the reasons described above, we adopted a cuff-based blood pressure measuring method for the development presented below. Although we had developed small, handy wrist blood pressure monitors, the wrist wrapping part, including the cuff, was too large to be worn all the time: even the narrowest one was 64 mm wide. To make the device wearable, the width of this wrapping part had to be reduced at least by half. The main unit also had to be reduced in size accordingly.
With the wrapping part set to a target width of 30 mm, the cuff cannot be more than approximately 25 mm wide to fit the wrist. An excessively narrow cuff width, however, would lead to, for example, insufficient pressing force applied to the wrist arteries or an extreme gap between the intra-cuff pressure and the actual pressure applied to the arteries, posing the problem of failure to take correct blood pressure measurements.
Reports have long existed to the effect that for accurate measurement, a cuff needs to have a width appropriate to the diameter of the arm or wrist to be pressed. According to, for example, Alexander et al., for sufficient transmission of its internal pressure to the arteries, a cuff needs to be at least 1.2 times wider than the diameter of the arm around which it is worn7) .
The cuff of our wrist blood pressure monitor had been reduced through the elaboration of its structure and material to a width of 52 mm for the maximum applicable wrist circumference of 215 mm and to approximately 0.8 times the maximum wrist diameter. For the development at issue here, we decided to go further and set a cuff width of 25 mm, less than half of the above width, as the development target. Considering that this challenge could not be solved through additional improvements to conventional structures, we decided that the compression structure and the pressure sensing method should be brought back to the drawing board.
Let us be more specific here. Conventionally, a single cuff was used to compress the wrist and arteries and to detect the applied pressure and the minute pressure oscillations transmitted from the arteries. We, however, decided that the wrist/artery compression function and the wrist-surface pressure detection function should be assigned to two separate cuffs (the compression cuff and the sensing cuff). Moreover, we came up with the idea of modifying the compression pressure sensing structure by the addition of a back sheet structure for optimizing the distribution of pressure transmitted to the arteries during compression. The next and subsequent chapters describe in detail the development of this cuff structure, which led to a successful reduction of the cuff width by more than a half without compromising the measurement accuracy.
2. The measuring principle of the blood pressure monitor and the cuff width issue
2.1縲Oscillometric method
Blood pressure measurement techniques fall broadly into two categories: direct arterial sphygmomanometry, in other words, arterial catheterization for direct measurement of blood pressure, and non-invasive sphygmomanometry in which a cuff applies external pressure that interacts with the intra-arterial pressure to induce a physical phenomenon used for non-invasive blood pressure estimation.
For home-use blood pressure monitors, the oscillometric method, a form of non-invasive sphygmomanometry, is in wide use. In the oscillometric method, the pressure in the cuff wrapped around the upper arm or the wrist (intra-cuff pressure) is raised to above the systolic blood pressure and then gradually lowered to detect the pressure oscillations to be superposed on the intra-cuff pressure. These pressure oscillations result from the intra-arterial pressure, in other words, the relationship between the blood pressure and the artery-tightening pressure. When the intra-cuff pressure falls below the systolic blood pressure, the occluded arteries start to open and close by small amounts. The area of the arterial opening increases with the decrease in the intra-cuff pressure. When the intra-cuff pressure further drops below the diastolic blood pressure, the arteries shift from an opened state to a slightly expanded state, showing a decreased change in the area of its opening. These changes in the cross-sections of the arteries are transmitted to the cuff to cause minute changes to the cuffハシs volume and minute oscillations of the intra-cuff pressure. Fig. 1 shows the relationship between the reduced pressure and the minute pressure oscillations.
The top panel of Fig. 1 shows minute pressure oscillations superposed on the intra-cuff pressure being reduced while the bottom panel shows a minute pressure oscillation waveform detected using a high pass filter. This change in the amplitude of the pulsating waveform allows the determination of the systolic and diastolic blood pressures. Some blood pressure monitors take measurements during increasing the pressure because similar data result from a gradual increase in the intra-cuff pressure.
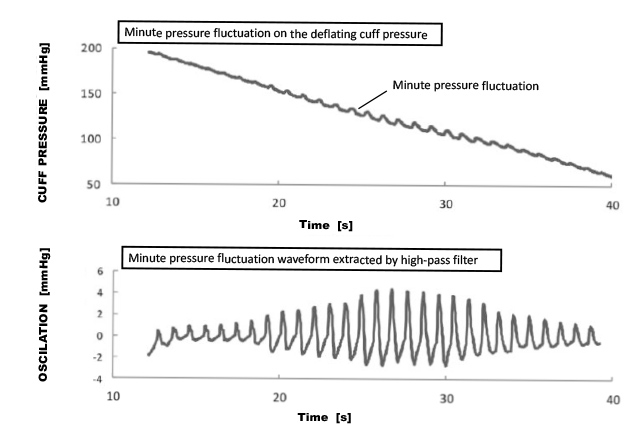
2.2縲Technical challenges to a narrow cuff width
The pressure used for blood pressure measurement is the intra-cuff pressure. Based on the assumption that the internal pressure of the compression cuff is substantially equal to the pressure on the arteries under compression pressure, the interaction between the intra-arterial and extra-arterial pressures is used to take blood pressure measurements.
A narrow cuff width leads to the following two challenges:
- (1) The cuff needs a higher internal pressure to occlude the arteries.
- (2) The robustness of the oscillations superposed on the cuff decreases.
The next subsection explains the causes of these challenges.
2.2.1縲Higher pressure required to occlude the arteries
As shown in Fig. 2, the inflation of the narrow width cuff is insufficient by an amount even under the same intra-cuff pressure
. This condition is the so-called insufficient stroke. For the aim to compensate for this shortfall
and occlude the arteries, the intra-cuff pressure must be further elevated to inflate the cuff.
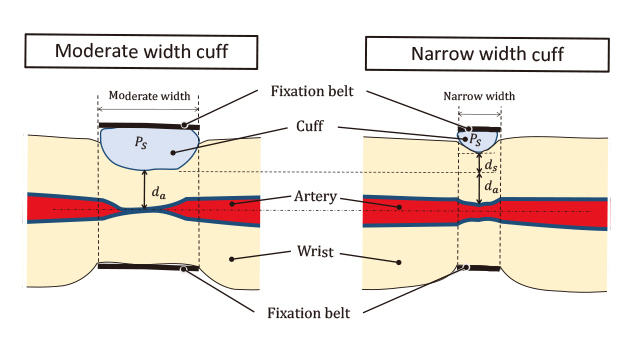
The difference between the cuffハシs internal pressure and its external pressure applied to the wrist (internal-external pressure difference) increases as the cuff inflates to a higher volume. Fig. 3 illustrates this relationship.
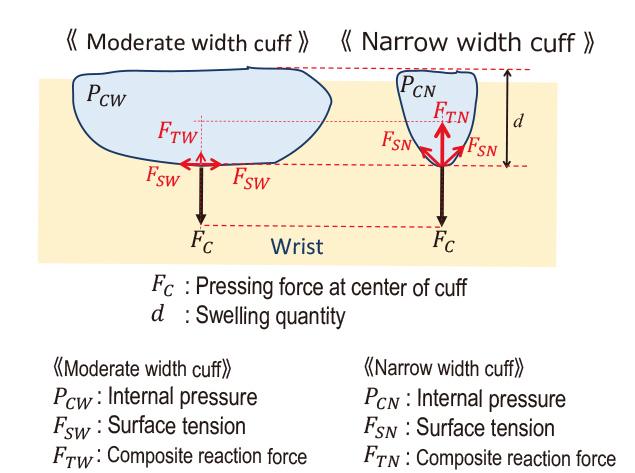
An increase in tension occurs when the cuff inflates and stretches the cuff sheet significantly ( <
). Besides, the inflation of the cuff causes an increase in the working angle, resulting in a remarkably large resultant force of tension (
竕、
). Therefore, even if the compression force
applied to the wrist is the same, the internal pressure of the narrow width cuff will be significantly larger than that of the proper width cuff (
竕、
).
2.2.2縲Decrease in the robustness of pulse wave oscillations
With a proper cuff width, the resulting pressure distribution curve has a flat top around the arteries as shown in Fig. 4. Meanwhile, the use of a narrow width cuff results in a mountain-shaped distribution curve without a flat top.
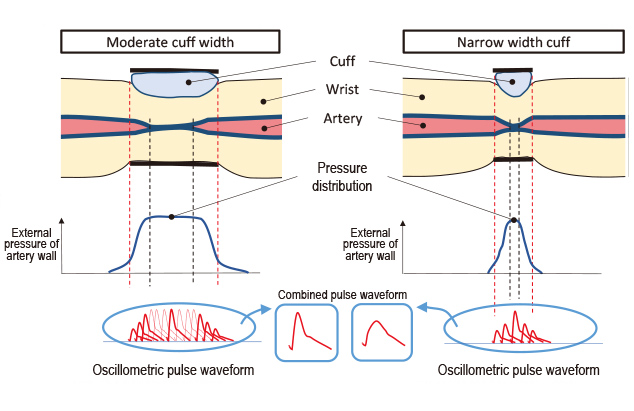
The difference between the pulsing blood pressure and the extra-arterial pressure causes an arterial volumetric change that, in turn, oscillates the volume of the cuff, resulting in a cuff pressure pulse wave superposed on the intra-cuff pressure. When pressure is not uniformly distributed over the external wall of the arteries, a non-uniform synthesized volumetric change is detected as a cuff pressure pulse wave, the concept of which is shown by the synthesized waveforms in Fig. 4. In the case of the narrow width cuff, the proportion of the pre-synthesis main waveforms is low. Hence, it is surmised that a considerably blurry shape will result from the waveforms in the center region. The appearance of a pressure distribution depends on the physical features of each person, such as wrist circumference, wrist bone shape, and muscle quality. The resulting error trend curve differs from person to person, resulting in significant variations. Slight differences in the as-worn position of the cuff also cause changes to this pressure distribution, resulting in reduced reproducibility.
3. Realization of a wearable blood pressure monitor
3.1縲Outward appearance and cuff structure of the device
The previous chapter explained the challenges expected to result from a reduced cuff width. To realize a blood pressure monitor wearable all the time, however, we considered it necessary to equip a device of a size and design similar to a wristwatch with the function of a blood pressure gauge. Fig. 5 shows the HeartGuideTM wearable blood pressure monitor, the product featuring the cuff structure we developed this time as worn around the wrist.
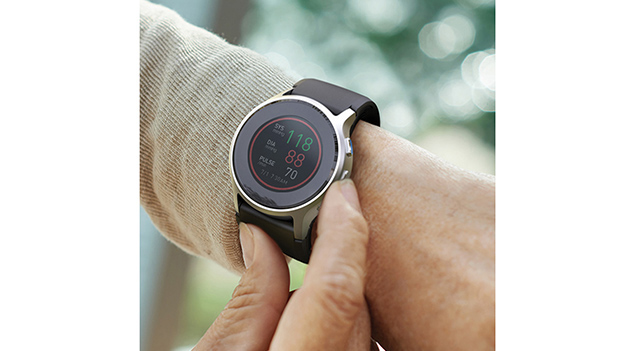
This product has a 30 mm-wide belt with a 25 mm-wide cuff on its inner side. With its width narrowed so much, the device is wearable around the wrist all the time, just like a wristwatch. Fig. 6 shows a cross-sectional view of the cuff structure and the wrist with this blood pressure monitor worn as shown and in measuring action. The main body equipped with a control display is on the dorsal side of the wrist shown at the bottom of the figure. The cuff structure part is wrapped and worn around the wrist by fastening the belt fixed to both ends of the main body onto the upper palmar side of the wrist.
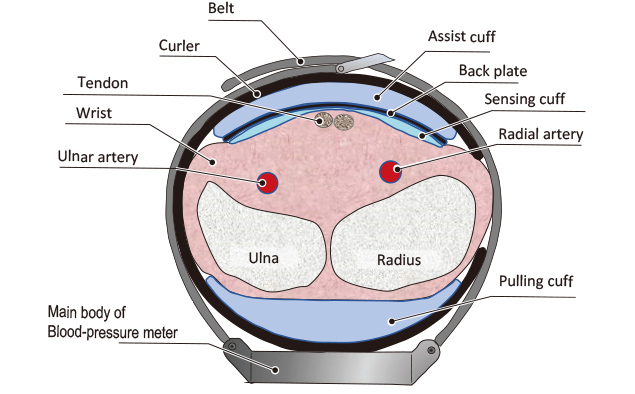
The cuff structure part is fixed to the curler on the inner side of the belt for easier positioning on the wrist. An assist cuff, a back sheet, and a sensing cuff are arranged in the order listed from top to bottom while a tension cuff is provided on the dorsal side of the wrist. The sensing cuff is positioned and worn to cover the palmar side of the wrist for blood pressure measurement.
For blood pressure measurement, the tension cuff and the assist cuff expand to squeeze the wrist between from front and back to compress it gradually. The sensing cuff detects the pressure applied to the wrist surface and the minute pressure oscillations superposed on that pressure. These minute pressure oscillations result from the transmission of the opening and closing vibrations in the radial and ulnar arteries to the sensing cuff: the opening and closing vibrations vary depending on the relationship between the blood pressure pulsations in both arteries and the pressure around the arteries. The back sheet works to give as flat a pressure distribution as possible to the arteries.
3.2縲Reducing the compression cuff width
Fig. 6 shows the structure of an actual product, whose tension and assist cuffs expand to compress the wrist and the radial and ulnar arteries from above and below. For the sake of explanatory simplicity, we consider here a structure in which a single compression cuff compresses the wrist, as shown in Fig. 7. The compression cuff must meet the requirement that it should give a flat pressure distribution to compress and occlude the arteries without giving rise to the robustness issue discussed in Subsection 2.2.2 above.
For a flat compression pressure distribution for compressing the arteries, the wrist surface must be compressed flatly. Therefore, we decided to introduce a back sheet to give a flat compression surface. Fig. 7 shows the changes in pressure distribution for with and without the back sheet.
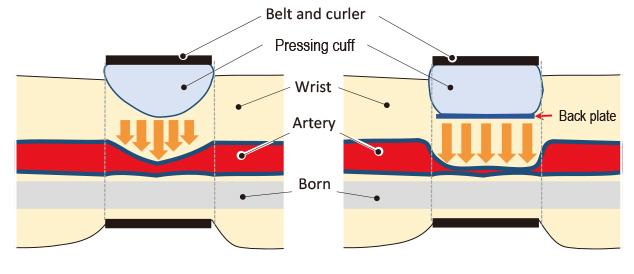
3.3縲Forming a flat compression pressure distribution by the back sheet
To determine first if a flat compression pressure distribution could be given, we simulated the distribution of pressure on the arteries using a computer-aided engineering (CAE) analysis program. Fig. 8 shows the results of simulating the distributions of the periarterial pressure and the skin surface pressure for three cuff widths as the internal pressure of the compression cuff was raised to 300 mmHg.
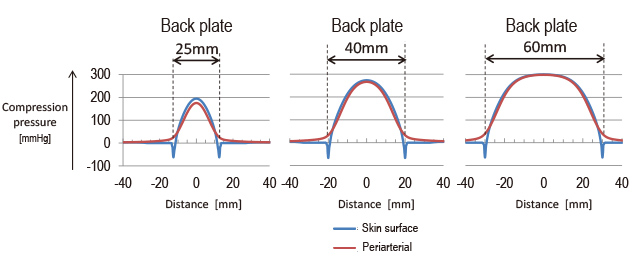
The 60 mm cuff width (right) was a condition similar to the cuff width of the existing wrist blood pressure monitor. The 25 mm cuff width (left) was the target condition set this time. The 40 mm cuff width was added as an intermediate condition.
With the 60 mm cuff width, the peak of the pressure distribution nearly coincided with the compression pressure of 300 mmHg, and a somewhat flat part appeared near the peak. With the two other narrower cuff width conditions, even the maximum pressure portion failed to reach 300 mmHg. The results of this simulation were exactly to the content of Subsection 2.2.1 above. Then, another simulation was performed of the periarterial pressure and the skin surface pressure, as the internal pressure of the 25 mm-wide cuff was raised to 300 mmHg with the back sheet affixed to the cuff. The results were as shown in Fig. 9.
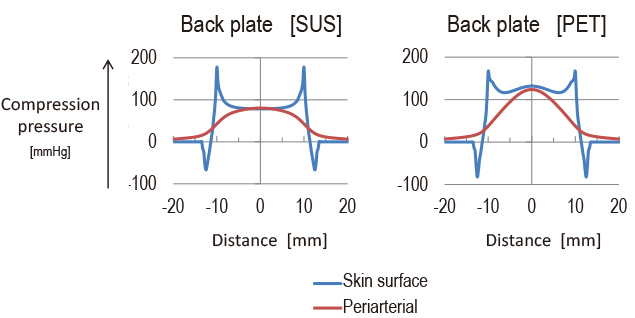
The conditions for the back sheet were a 0.15 mm-thick metal sheet (stainless steel) and a 0.35 mm thick PET resin sheet. The simulation results showed that a flat distribution of periarterial pressure appeared only with the metal (stainless steel) back sheet. Probably, the PET resin sheet bent and failed to apply flat pressure to the wrist surface proper.
These results, however, confirmed that a flat pressure distribution was controllable to some extent by the sheet hardness in the axial direction. Accordingly, we decided to obtain a compression structure by manufacturing a back sheet of a structure resistant to bending in the axial direction of the wrist and flexibly adjusted to the circumferentially curved surface of the wrist and affixing the back sheet to the compression cuff.
Under whichever condition, the periarterial pressure was around 100 mmHg, considerably lower than the compression pressure of 300 mmHg. This fact means that a narrower cuff width leads to a reduced ultimate pressure to the arteries, as explained above, and that the addition of a back sheet likely leads to a further reduced ultimate pressure.
3.4縲Compression cuff-plus-sensing cuff structure
The addition and adjustment of the back sheet led to the realization of the compression structure shown on the right panel of Fig. 7 in Section 3.2. Then, an experiment was performed to determine the magnitude of the difference that would occur between the pressure on the arteries and the compression cuff pressure when this compression structure compressed a real human wrist.
Fig. 10 shows how the experiment went. As the wrist of a subject with an systolic blood pressure of 110 mmHg was gradually compressed, the oscillations of the filtered pulse wave almost completely disappeared after the elapsed time of about 22 seconds. The filtered pulse wave was obtained as an extraction of a pulsation resulting from the relationship between the arterial pressure oscillation and the periarterial pressure from the lower to the systolic blood pressure per pulse stroke. When a pressure slightly higher than the systolic blood pressure of 110 mmHg was applied around the arteries of the subject, the arteries were occluded to stop the oscillations of the filtered pulse wave. The internal pressure of the compression cuff at the elapsed time of 22 seconds was approximately 255 mmHg. In other words, a pressure difference of approximately 145 mmHg occurred relative to the pressure transmitted to the arteries. This pressure difference was the real one resulting from a reduced cuff width and the addition of the back sheet as explained above in Section 3.3.
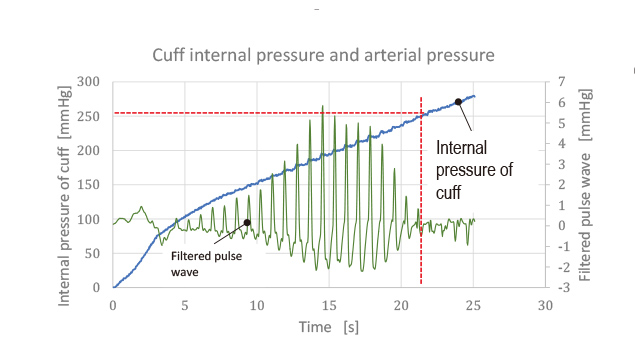
The internal pressure of the compression cuff differed excessively from the periarterial pressure: the value of the intra-cuff pressure was inappropriate for use in the blood pressure determination. It followed, then, that the pressure applied around the arteries should be measured by a different method. Hence, we considered the idea of a pressure sensor placed in between the back sheet and the wrist surface for pressure detection.
The above-mentioned pressure sensor may be a multiple number of surface pressure sensors or strain gauges. The wrist, however, includes hard parts, such as tendons and bones, which come under the pressure-sensing surface. These anatomical parts exert a strong reaction force against an applied compression pressure, resulting in a non-uniform distribution of pressure. It takes a complex 3D analysis, and is unrealistic, to estimate from this pressure distribution the pressure applied to the arteries.
Strain gauges are not an option either: it is difficult for a strain gauge to provide a pressure resolution and accuracy sufficient for blood pressure measurement under a back sheet flexibly bendable at the timing of wrist compression because the sensitivity and accuracy of the strain gauge depend on the strain body to which it is affixed.
Then, we came up with an idea of heat welding the circumferences of two thin sheets to form them into a sensing cuff of a bag-like structure. Figs. 11 show how a sensing cuff filled with a minute amount of fluid is pushed against a living body part by the compression cuff via the back sheet.
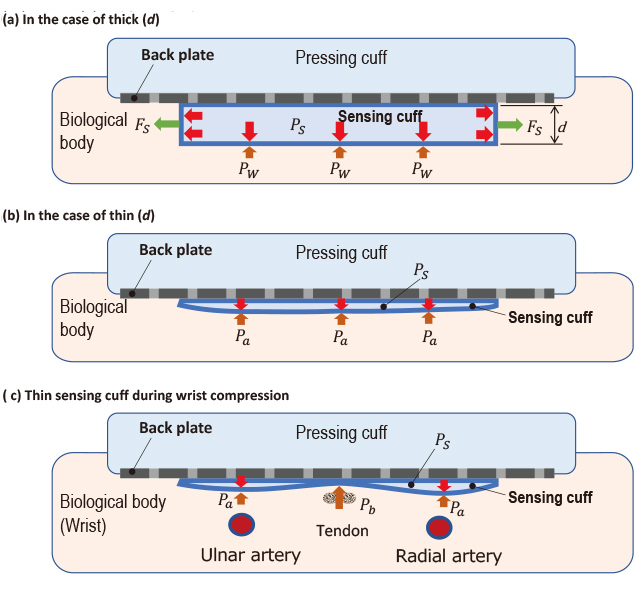
In Figs. 11 (a) to (c), the internal pressure of the sensing cuff is . Fig. 11(a) shows a case where the walls to the right and left of the sensing cuff have a large thickness (thickness
). In this case, the lateral force
caused by the internal pressure
pulls the top and bottom sheets of the sensing cuff to the right and left, so that the surface pressure of the living body part,
, and the internal pressure of the sensing cuff,
, are in a relationship expressed as
>
. This relationship means that the difference between the two pressures can be minimized by reducing the wall height
infinitely.
Fig. 11(b) shows the living body part compressed by the sensing cuff with reduced almost to zero. In this case,
竕
holds because almost no lateral tensile force occurs. Then, Fig. 11(c) schematically shows a cross-sectional view of the wrist compressed using the structure shown in Fig. 11(b). The wrist is shown along with the tendons and the radial and ulnar arteries. Usually, the hard tendons have tension and hence exert a strong reaction force
against an applied compression pressure to flatten the sensing cuff to a degree. The displaced fluid gathers in the relatively soft tissues above the two arteries so that the internal pressure
and the skin surface pressure
come into balance with each other. When the amount of fluid is minute and the cuff expands only slightly,
竕
holds. Assuming then that the living body part is compressed vertically, the skin surface pressure
can be regarded as substantially equal to the pressure applied around the arterial walls. Therefore, the internal pressure of the sensing cuff,
, is expected to be quite close to the pressure applied to the arterial walls.
Preferably, the minute amount of fluid filled into the sensing cuff should consist of incompressible material and have a low viscosity to avoid obstructing the transmission of arterial oscillations. It is, however, realistically impractical to keep a constant minute amount of fluid, such as water, in the sensing cuff. Accordingly, we chose to refill the sensing cuff with a certain amount of air, though compressible but controllable by the pump or valve of the blood pressure monitor, as required for each measurement. The amount of air in the sensing cuff affects the pressure detection sensitivity and relates directly to the measurement accuracy. Therefore, many considerations had to be made to ensure the robustness and optimization of the amount of the refill. We, however, refrain here from explaining in detail.
3.5縲Creases and bends in the back sheet and the sensing cuff
Then, when the intra-cuff pressure was raised much higher to measure the blood pressure of a person with hypertension, a phenomenon occurred of an increase in the variability of blood pressure measurement errors. We considered that this phenomenon had resulted from bends or creases that occurred as a result of the additional pressure increase that caused further compression of the wrist, resulting in a reduced creepage distance along the area on which the back sheet and the sensing cuff were placed when the device was worn around the wrist.
Fig. 12 shows an X-ray CT image of a sensing cuff bent under a pressure of approximately 300 mmHg when a wrist simulator was compressed.
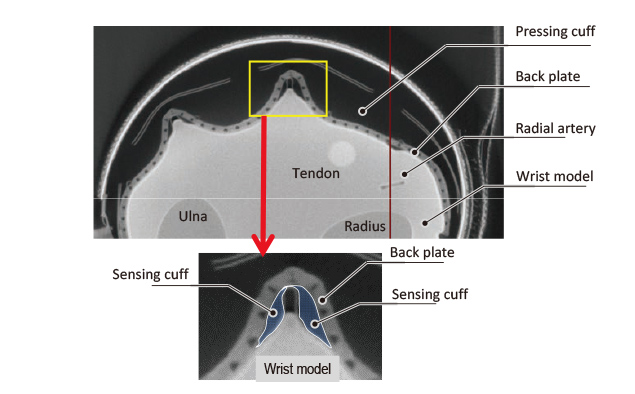
The sensing cuff and the back sheet were bent and protruded to look as if they were gripping the wrist simulator. Air gathered toward both ends of the sensing cuff. Neither portion faced straight to the wrist surface. The resulting pressure had no direct relevance to the blood pressure and was hence considered to have caused large and varied measurement errors. For correct detection of the compression pressure applied to the wrist surface, the back sheet and the sensing cuff need to remain unstretched and crease-free when pushed against the wrist.
3.6縲Solving the bend problem with a tension cuff
Then, we considered a method of compressing the wrist by pulling the back sheet and the sensing cuff in the as-worn state of the device. For the realization of this method, a pulling cuff (tension cuff) was provided on the dorsal side of the wrist to use the expansion of this cuff for arterial compression.
This tensile structure allowed the sensing cuff to fit unstretched and crease-free on the palmar surface of the wrist and compress the wrist properly. Fig. 13 shows the improved arterial compression method.
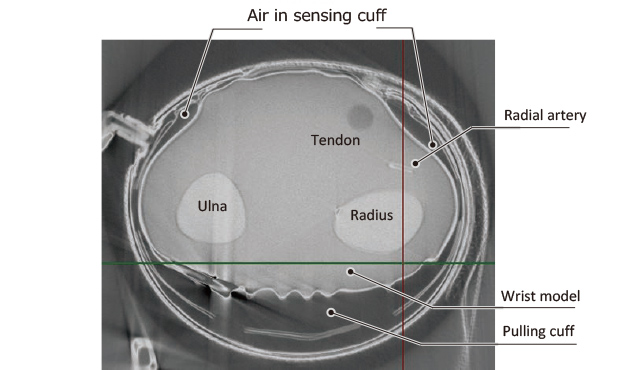
The actual product is also equipped with an assist cuff on the outer side of the back sheet as an aid in providing improved wrist compression efficiency and usability.
3.7縲Realization of a compression structure based on a narrow width cuff
It was difficult for a conventional wrist-worn blood pressure monitor to meet the required blood pressure measurement accuracy for approval as a medical device unless its cuff width was 52 mm or more.
The narrow-width compression structure developed this time using a back sheet, a sensing cuff, and a tension cuff, among other parts, provides sufficient performance in wrist arterial compression and pressure sensing despite its small cuff width of approximately 25 mm. Thus, the intended blood pressure monitor wearable around the wrist all the time just like a wristwatch to take measurements at any time was successfully brought into commercial production.
The next chapter discusses the blood pressure measurement accuracy and usability of the wearable blood pressure monitor and outlines its product specifications.
4. Measurement accuracy and usability of the watch-type blood pressure monitor
In January 2019, we launched the HeartGuideTM, which is a watch-type wearable blood pressure monitor approved as a medical device by the United States Food and Drug Administration (USFDA). This device monitors continuously varying blood pressure oscillations to allow a good understanding of hypertension-related risks undetectable by physical exam alone and the characteristics of blood pressure oscillations that differ from person to person.
4.1縲Measurement accuracy
This blood pressure monitor meets the clinical measurement accuracy required in ANSI/AMMI/ISO 81060-2:2013, the US-adopted clinical test standard for automatic non-invasive sphygmomanometry. Table 1 shows the results of the clinical accuracy test. A paper on this watch-type wearable blood pressure monitor appeared in the Journal of Clinical Hypertension8) to discuss and demonstrate its measurement accuracy.
Validation for HEM-6410T-ZM (M size for US market) |
Validation for HEM-6410T-ZL (L size for US market) |
|
---|---|---|
Difference of SBP for criterion1,mmHg | - 0.9ツア7.6シpassedシ | 2.4ツア7.3シpassedシ |
Difference of SBP for criterion2,mmHg | - 0.9ツア6.8シpassedシ | 2.4ツア6.5シpassedシ |
Difference of DBP for criterion1,mmHg | - 1.1ツア6.1シpassedシ | 0.7ツア7.0シpassedシ |
Difference of DBP for criterion2,mmHg | - 1.1ツア5.5シpassedシ | 0.7ツア6.5シpassedシ |
Date are expressed as meansツアstandard deviations.
Besides, a comparative blood pressure measurement test between a 24-hour ambulatory blood pressure monitoring (ABPM) device and the HeartGuideTM wearable blood pressure monitor was performed for all hours of the day except night hours. The measurements by the HeartGuideTM wearable blood pressure monitor were equivalent to those by the ABPM, the standard for daytime blood pressure measurement, thus showing the potential for enhancing the reliability of hypertension diagnosis and treatment9) .
4.2縲Product specifications
Fig. 14 shows the outward appearance of the product, while Table 2 shows the main specifications of the product. The width of the band is 30 mm, less than half of the 64 mm in the conventional model.
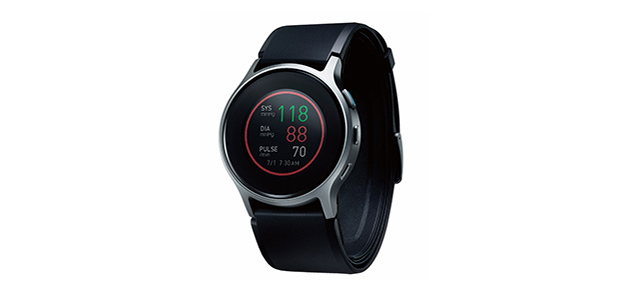
Model | BP8000-Mシ HEM-6410T-ZMシ |
---|---|
Dimensions | Diameter approximately 48 mm, Case thickness approximately 14 mm, Band width approximately 30 mm |
Weight | Approximately 115 g |
Display | Transflective memory-in-pixel LCD |
Measurable wrist circumference | 160 to 190 mm |
Battery life | Two days (measurements eight times/day) |
5. Conclusions
A narrow cuff width leads to the need for higher pressure to occlude the arteries and thus causes instability in pulse waveform detection, thereby posing the problem of reduced blood pressure measurement accuracy. The conventional model used a single cuff to compress the wrist and gradually occlude the arteries and to detect the intra-cuff pressure accompanied by minute pressure oscillations induced in response to arterial blood pressure pulsations. As a solution to the above challenge, a structure equipped with two separate cuffs, one intended for wrist compression and the other for wrist compression pressure detection, was adopted to achieve a more than one-half reduction in the width of the band from 64 mm in the conventional model to 30 mm. Although not mentioned above in this paper, the main body size was also reduced to approximately one-third that of the conventional model to realize the watch-type wearable blood pressure monitor presented above herein.
OMRON HEALTHCARE Co., Ltd., is committed to the challenges of providing higher accessibility to blood pressure measurements, providing more opportunities to detect dangerous blood pressure oscillations and predict disease risks, and achieving zero occurrences of cerebral and cardiovascular diseases (zero events). The watch-type wearable blood pressure monitor developed this time is the first step in this direction. We will further reduce the size of the device, increase wearability for more users, and enable night-hour measurement during sleep to make blood pressure monitoring available to anyone, anywhere, at any time towards the achievement of zero events.
References
- 1)
- H. Kubota, 窶廩istory of blood pressure,窶 Jpn. J. Med. Instrum., vol. 80, no. 6, pp. 615 窶 621, 2010.
- 2)
- O. Shirasaki, 窶彝oles and advancements of blood pressure monitors in cardiovascular medicine,窶 Jpn. J. Med. Instrum., vol. 80, no. 6, pp. 622 窶 631, 2010.
- 3)
- Y. Imai, T. Okubo, M. Kikuya, and J. Hashimoto, 窶廣pplication of Home Blood Pressure Measurement to Clinical Practices,窶 (in Japanese), J. Jpn. Soc. Intern. Med., vol. 93, no. 9, pp. 202 窶 209, 2004.
- 4)
- E. Rapsomaniki, A. Timmis, J. George, M. Pujades-Rodriguez, A. D. Shah, S. Denaxas, et al., 窶廝lood pressure and incidence of twelve cardiovascular diseases: Lifetime risks, healthy life-years lost, and age-specific associations in 1ツキ25 million people,窶 Lancet, vol. 383, no. 9932, pp. 1899 窶 1911, May 31 2014.
- 5)
- T. G. Pickering, 窶弋he 9th Sir George Pickering memorial lecture. Ambulatory monitoring and the definition of hypertension,窶 J. Hypertens., vol. 10, no. 5, pp. 401 窶 409, May 1992.
- 6)
- JSH Hypertension Management Guideline Development Committee, Guidelines for the Management of Hypertension 2014 [JSH2014], Non-Profit Organization the Japanese Society of Hypertension (in Japanese), 2014, p. 15.
- 7)
- H. Alexander, M. L. Cohen, and L. Steinfeld, 窶廚riteria in the choice of an occluding cuff for the indirect measurement blood pressure,窶 Med. Biol. Eng. Comput., vol. 15, no. 1, pp. 2 窶 10, Jan. 1977.
- 8)
- M. Kuwabara, K. Harada, Y. Hishiki, and K. Kario, 窶弖alidation of two watch-type wearable blood pressure monitors according to the ANSI/AAMI/ISO81060-2:2013 guidelines: Omron HEM- 6410T-ZM and HEM-6410T-ZL,窶 J. Clin. Hypertens. (Greenwich), vol. 21, no. 6, pp. 853 窶 858, Jun. 2019.
- 9)
- K. Kario, D. Shimbo, N. Tomitani, H. Kanegae, J. E. Schwartz, and B. Williams, 窶弋he first study comparing a wearable watch-type blood pressure monitor with a conventional ambulatory blood pressure monitor on in-office and out-of-office settings,窶 J. Clin. Hypertens. (Greenwich), vol. 22, no. 2, pp. 135 窶 141, Feb. 2020.
The names of products in the text may be trademarks of each company.